Amplifier Power Scaling
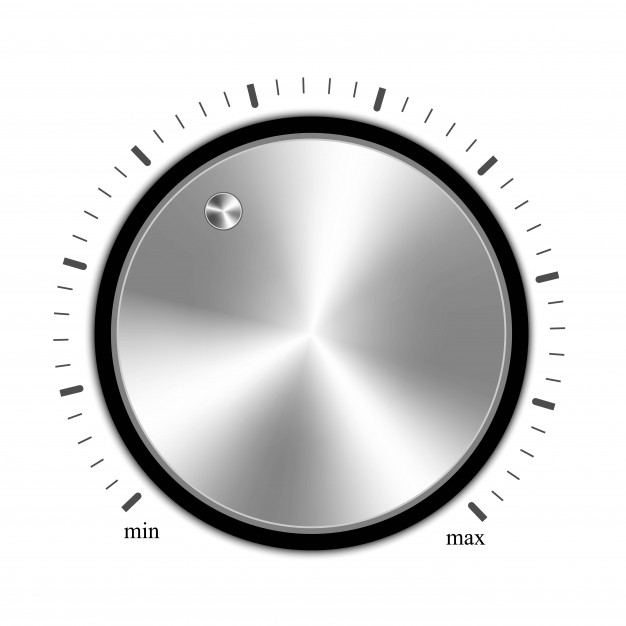
Overview
Power Scaling, in the context of guitar amplifiers, is a method by which the overall volume can be reduced without impacting the saturated tone, harmonics and dynamics of a fully cranked amp. Power scaling can be applied to the power section, the whole amp or any stage in between depending
on the requirement. Unlike a master volume, where part of the signal is sent to ground or two out of phase signals are mixed to cancel each other out, power scaling controls the amount of voltage which is sent to the plates (anodes), screens (where applicable) and grids of the
section being scaled. This will result in a decrease in current flow and decrease in power output i.e. watts.
Power scaling has been around for many years and has been implemented in different forms. In more recent times people such as Kevin O'Connor of London Power, Dave Zimmerman, Dana and Richie Hall of Hall Amplification have come up with different ways to modify power supplies in guitar amps to make the power output variable.
They have a number of different types of implementation based on the amp requirements and it's type of biasing i.e. cathode vs fixed. More information about Kevin O'Connor work with power scaling can be found here. Power scaling designs and kits have been given names such as VVR, VCB and VRM but equate to the same overall requirement of controlling high voltages via the combination of components such as MOSFET and potentiometers.
How Power Scaling Works
Power scaling requires that the voltage supply to an amplification stage of an amplifier is reduced. The key is to make sure that
all voltage requirements for the amplification stage (plate, screens and grids in the case of tubes) are reduced by the same ratio so as to not impact the characterstics of the audio signal being amplified. If the bias voltage were to remain the same during voltage reduction
then this would result in making the bias colder and therefore
the audio signal would breakup earlier and the tone will change.
One characteristic that will be impacted during power scaling is sag. This will occur due to the fact that there are reduced demands on the power supply during lower
voltage operation.
Another characteristic which occurs when the power is decreased is related to the levels of lows, mids and highs you hear as a listener. This has nothing to do with the circuit but more a case of the behaviour of human hearing.
At low listening volumes mid range is more prominent while low and high frequency ranges go into the background. At higher listening volumes the lows and highs sound more prominent whereas the mid range is softer. When dialing down the power in an amplifier you can compensate for these changes
by increasing the bass and treble via EQ knobs. Alternatively you could implement some form of compensation switch which boosts lows and highs.
The diagram below provides a visual representation of how a reduction in voltage for a pentode power tube will result in power output reduction. In this example anode (plate), screen and cathode voltages are being reduced by the same ratio.
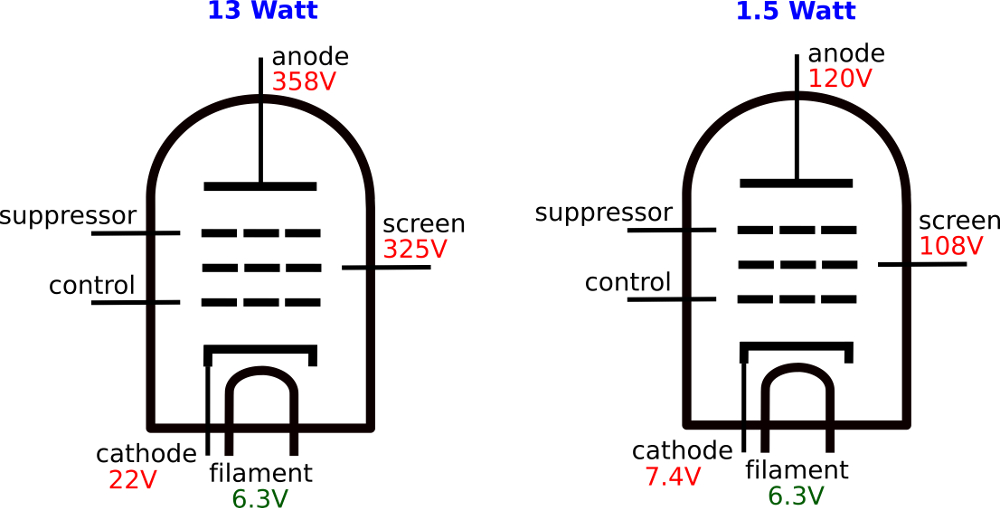
The table below provides some details with regards to voltage, current, resistance and power output for the above example. These rough calculations are based on the power stage being cathode biased rather than fixed bias.
Metric | Full Voltage | Reduced Voltage |
---|---|---|
Anode (V) | 358 | 120 |
Cathode (V) | 22 | 7.4 |
Cathode (Ω) | 570 | 570 |
Current (V) | 358 | 120 |
Power (W) | 13 | 1.5 |
How to Implement
Power scaling can be applied using fixed switching between different voltages or via a variable method that provides voltages between two fixed points. Power scaling can be applied to power amp, full amp or any stage in between depending on the application.
Fixed Switching | Variable |
---|---|
Fixed switching between voltages is typically accommplished by using a power transformer with muli-tap HT secondaries. Take an example of a power transformer with 0V - 120V - 275V secondaries. The 0V and 120V pairing provides 120V and the 0 and 275V pairing provides 275V. Using a toggle switch it is possible to switch between 120V and 275V B+ voltage supply. | Variable voltage supply typically makes use of MOSFETs to vary voltage output. By feeding the B+ voltage into an N-CHANNEL MOSFET drain, controlling the gate voltage by a potentiometer it is possible to vary the voltage level that exits the source. |
Fixed Switching
The diagram below provides a simplistic representation of a switched B+ voltage supply. If the transformer had more secondaries or there was more complex switching then there would be more possible voltage pairings and outputs. Please note that the switch used to toggle between different secondary ouput voltages will have to be a heavy duty high voltage rated AC type.
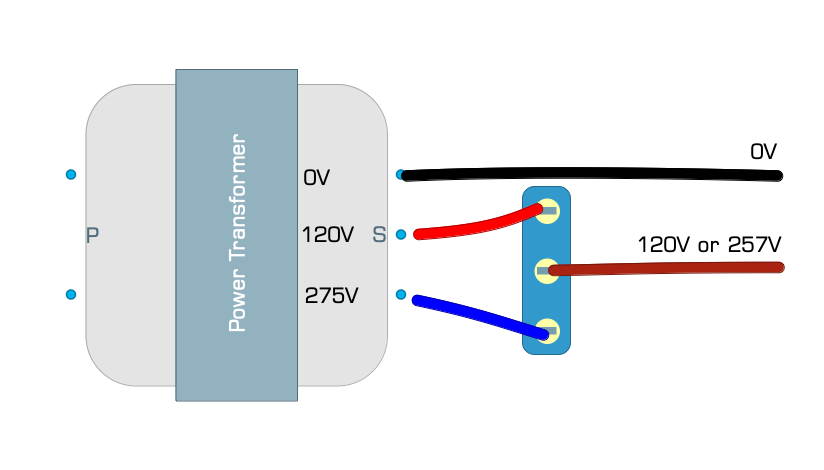
The following schematic provides an example implementation where switched power scaling is being applied to power tube section (120V vs 275V) and a separate bypass to preamp section is provided. Values of resistors and capacitors for filtering section will vary from amp to amp.
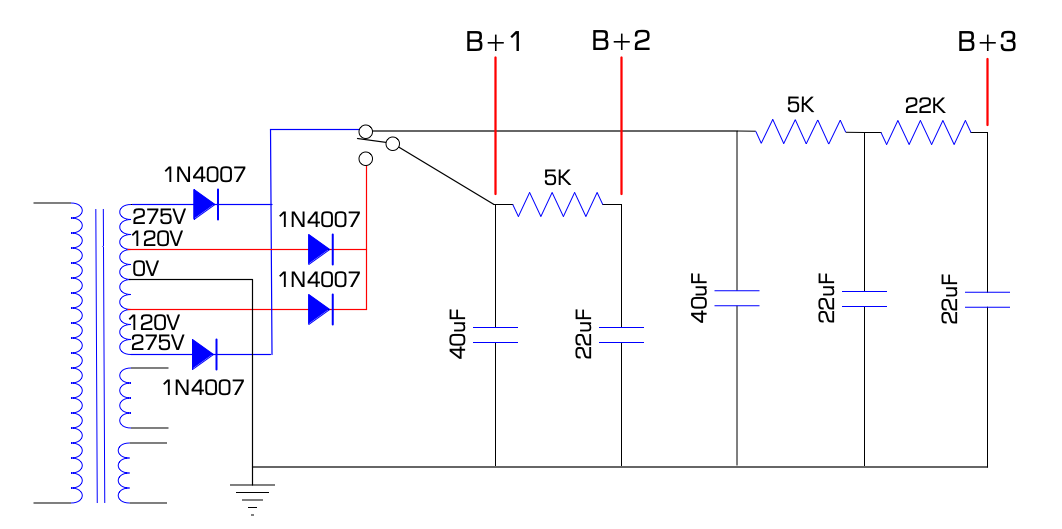
The following schematic provides the same example implementation but with power scaling applied to all amplification stages. Values of resistors and capacitors for filtering section will vary from amp to amp.
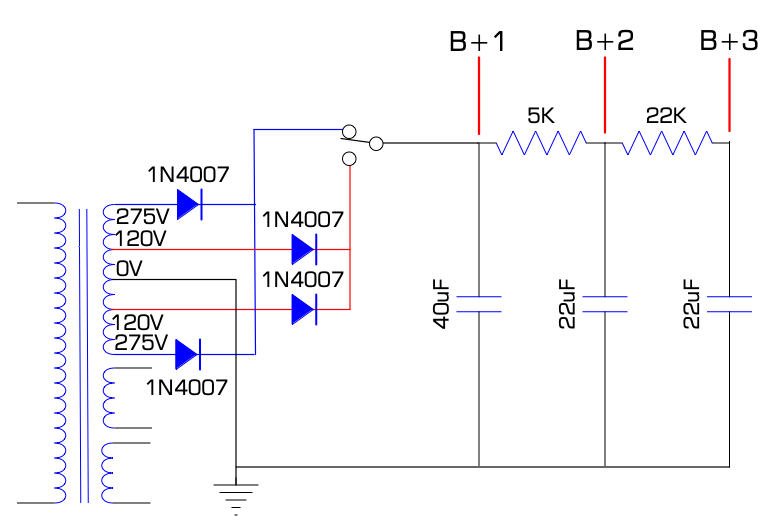
Variable Voltage
The diagram below provides a basic circuit required to provide a variable voltage supply in a cathode biased amplifier. There is no requirement to provide a separate tube bias voltage supply due to the fact that cathode biased tubes are self biasing i.e. a reduced current draw will result in a reduced voltage drop across the cathode resistor and a reduced bias voltage.
The key component used in variable voltage power scaling is the MOSFET (metal oxide semiconductor field effect transitor). MOSFETs are used as switches as well as voltage amplifiers. In power scaling, the switching functionality and transition between on and off, is being used to control the voltage and current flow between the source and drain terminals. When there is no voltage across the gate terminal, then the device does not conduct. When there is the maximum voltage across the gate terminal, then the device shows enhanced conductivity (enhancement mode).
In the simplest of forms of Power Scaling the power potentiometer changes the voltage supplied to the gate of the MOSFET and the B+ is channelled through the drain to source of the MOSFET. When the voltage supplied at the gate is low then the resistance is high between drain and source hence lower B+ voltage passing through. When the voltage supplied at the gate is high then the resistance is low between the drain and source hence higher B+ voltage passing through.
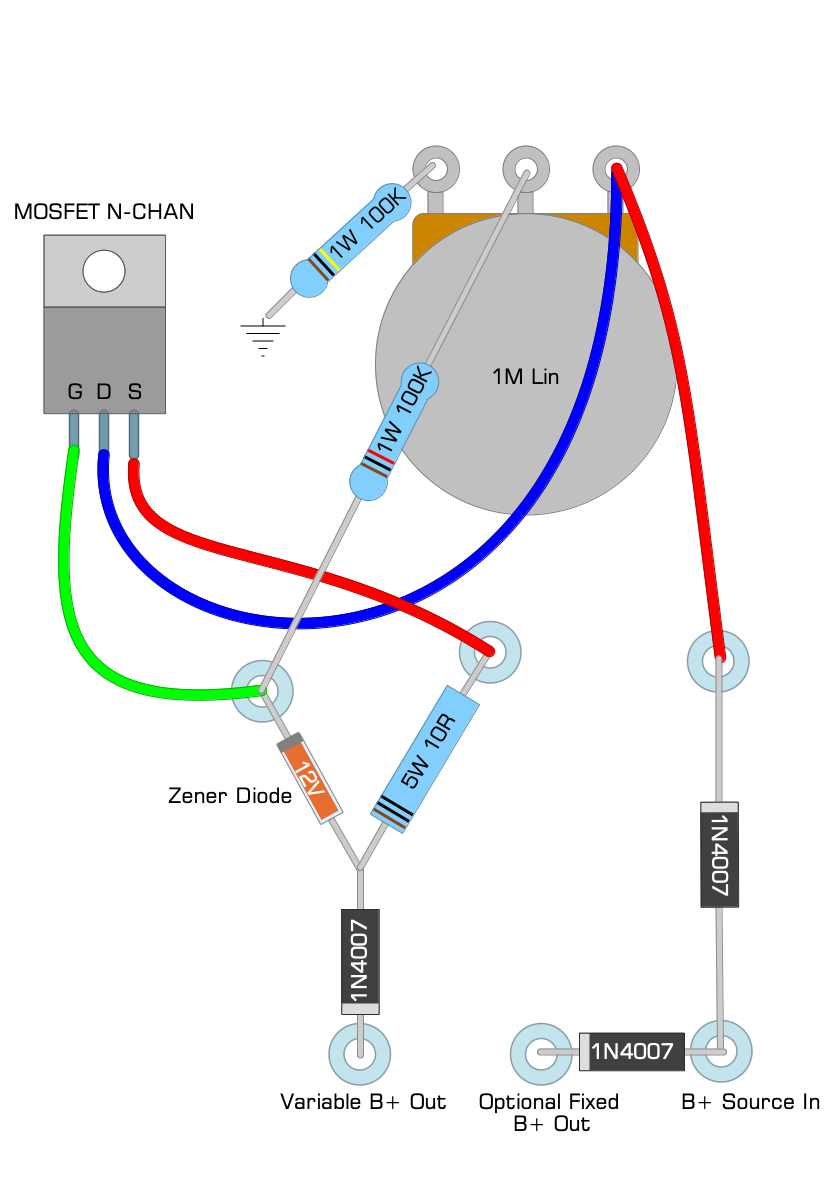
B+ Source: Rectified power source feeds into the circuit. The B+ source can be the rectifier output or first filter stage output.
Optional Fixed B+ Out: If only the power section is having power scaling then the preamp and phase inverter (if applicable and is optional) can be taken from this point.
Note that both B+ source and optional B+ have diodes to remove any possibility of circuit interaction.
Potentiometer: The potentiometer takes a feed from the B+ source and is used as a voltage divider.
Increasing the resistance decreases the voltage being fed to the MOSFET grid and results in lower voltage being sent to Variable B+. Either linear or audio taper pots can be used - test both to see how the sweep matches volume in the amp being scaled.
1W 100K resistor to ground: This sets the minimum voltage limit. If a lower voltage is required out of the power scaling circuit then this resistor should be reduced. To set the maximum voltage a resistor can be put in place prior to the
potentiometer.
1W 100K resistor centre lug: The resistor forms part of the current limiting circuit as well as being a gate stopper. The minimum resistance which can be used in R1 of voltage divider is 100K.
MOSFET: The mosfet has B+ in through drain, variable B+ out from source and control voltage through the grid. Increasing the grid voltage reduces the resistance between drain and source and as a result increased source voltage.
Zener Diode: The purpose of the Zener diode is to protect the MOSFET from over current. Under zero load the voltage on the MOSFET gate is similar to the source. As current increases through the mosfet this will cause
a voltage drop across the 5W 10R resistor (current limiting/sense resistor). If the voltage difference between either size of the zener diode exceeds 12V then zener starts conducting resulting in the gate voltage being reduced.
This will continue until the zener diode stops conducting. The values of the sensing resistor and zener diode vary with current requirements of the amp being scaled.
5W 10R: A component in the over current protection circuit. The purpose this resistor is to to cause a voltage drop during higher current flow. See "Zener Diode" for further details of over current protection.
Please note that there will be a certain amount of voltage drop due to 1) resistance between drain and source when fully open (RDSon of about 1 to 3 ohms) 2) resistor after the drain.
The following schematic provides an example implementation where power scaling is being applied to power tube section and a separate bypass to preamp section is provided. Values of resistors and capacitors for filtering section will vary from amp to amp. If using valve rectification then B+ source feeding the first 40uF filter capacitor will be the B+ output from the rectifier.
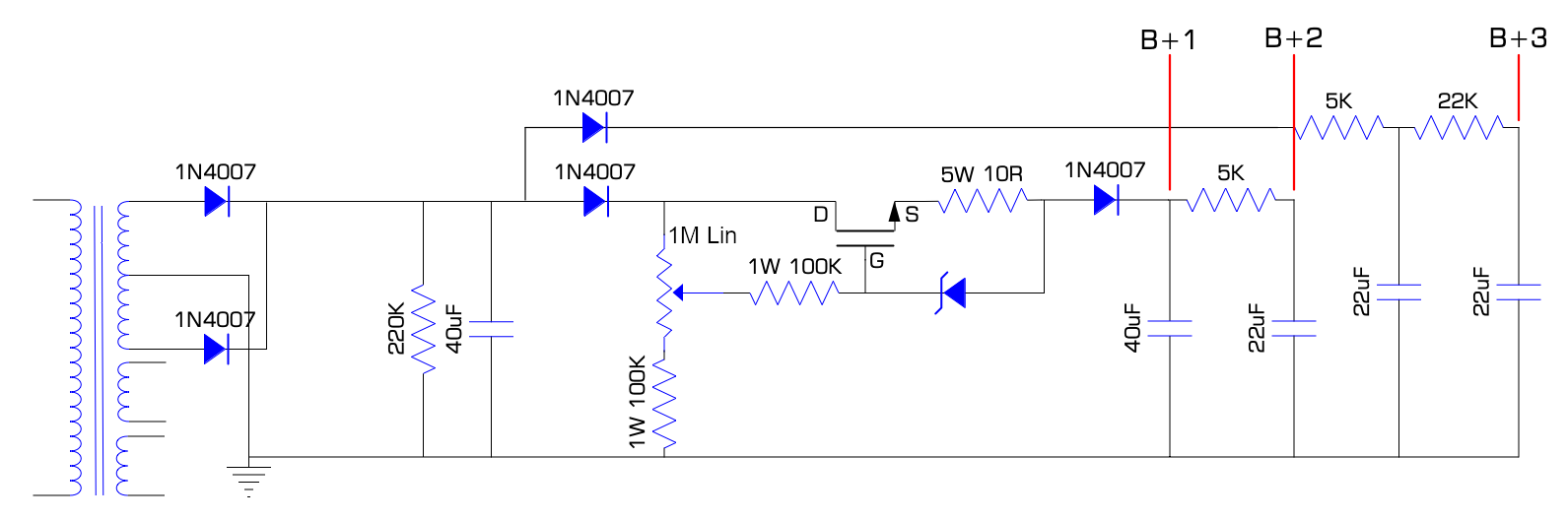
The following schematic provides the same example implementation but with power scaling applied to all amplification stages. Values of resistors and capacitors for filtering section will vary from amp to amp.
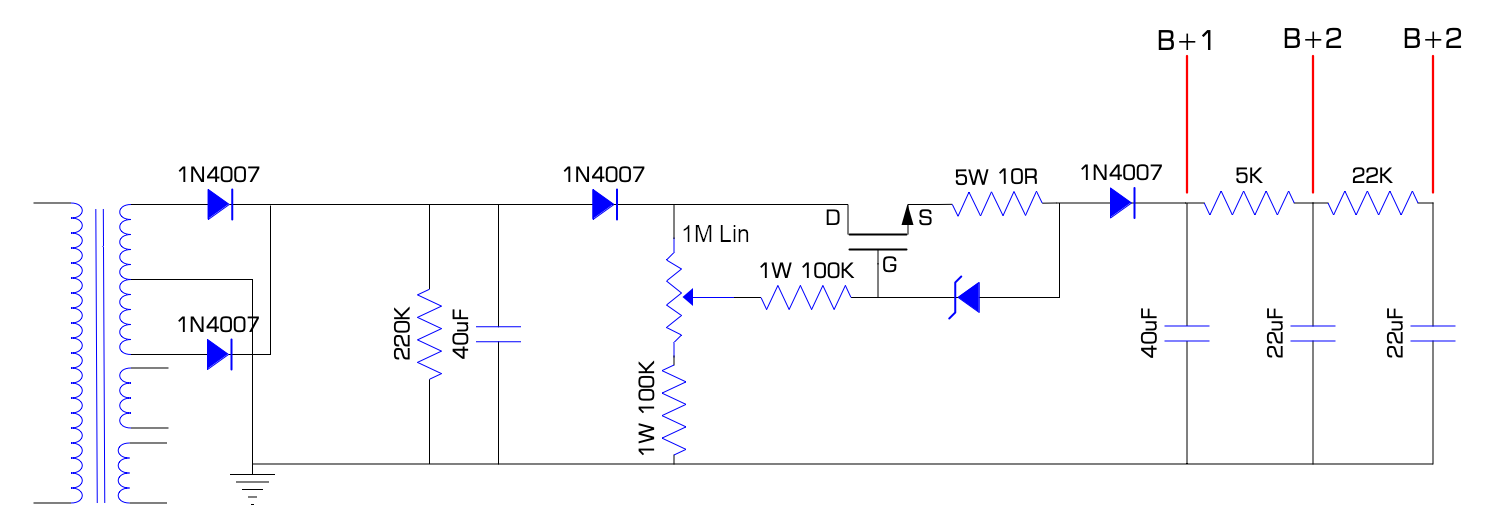
The following schematic highlights the purpose of each component or group of components in a basic cathode bias power scaling circuit.
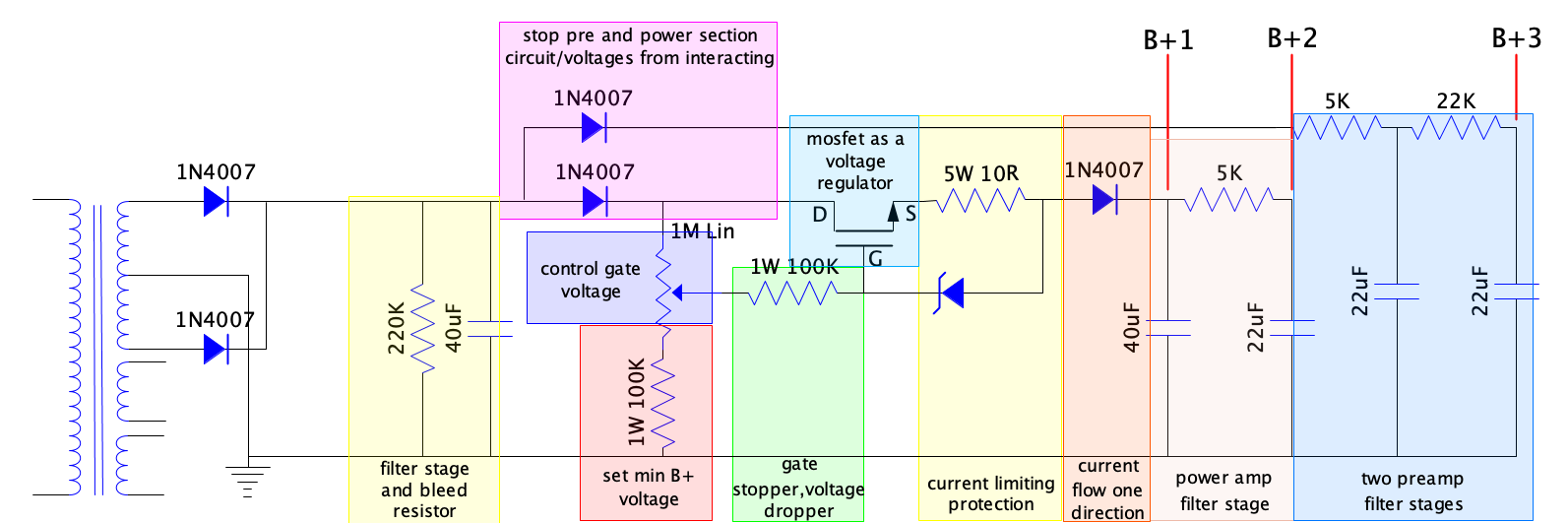
Power Scaling 101
This section provides general information about power scaling and reference material.
MOSFETS
In most circumstances MOSFETs will need to be secured to the chassis to dissipate heat. Screen only power scaling is a type which is likely not to need a heatsink for the MOSFET due to the small amount of current in the circuit. A chassis can be used to dissipate heat for implementions in amps between 5 to 30 watts. Any amp with greater wattage output should utilise a heat sink and thermal grease (thin layer). The MOSFET MUST be insulated from the chassis using a SIL-PAD or MICA insulator so that it does not short. The following order should apply: Mosfet > insulator > grease > chassis. In some cases there will be a requirement to use a nylon bolt and nut or insulated collar where the hole in the MOSFET is not inuslated.
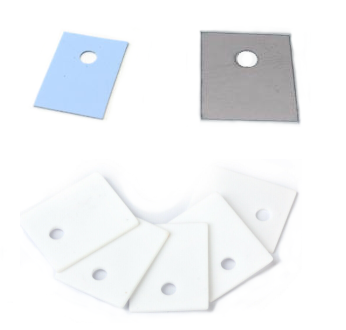
From top left: 1) silicone insulator 2) clear mica insulator 3) aluminium oxide or beryllium oxide insulator
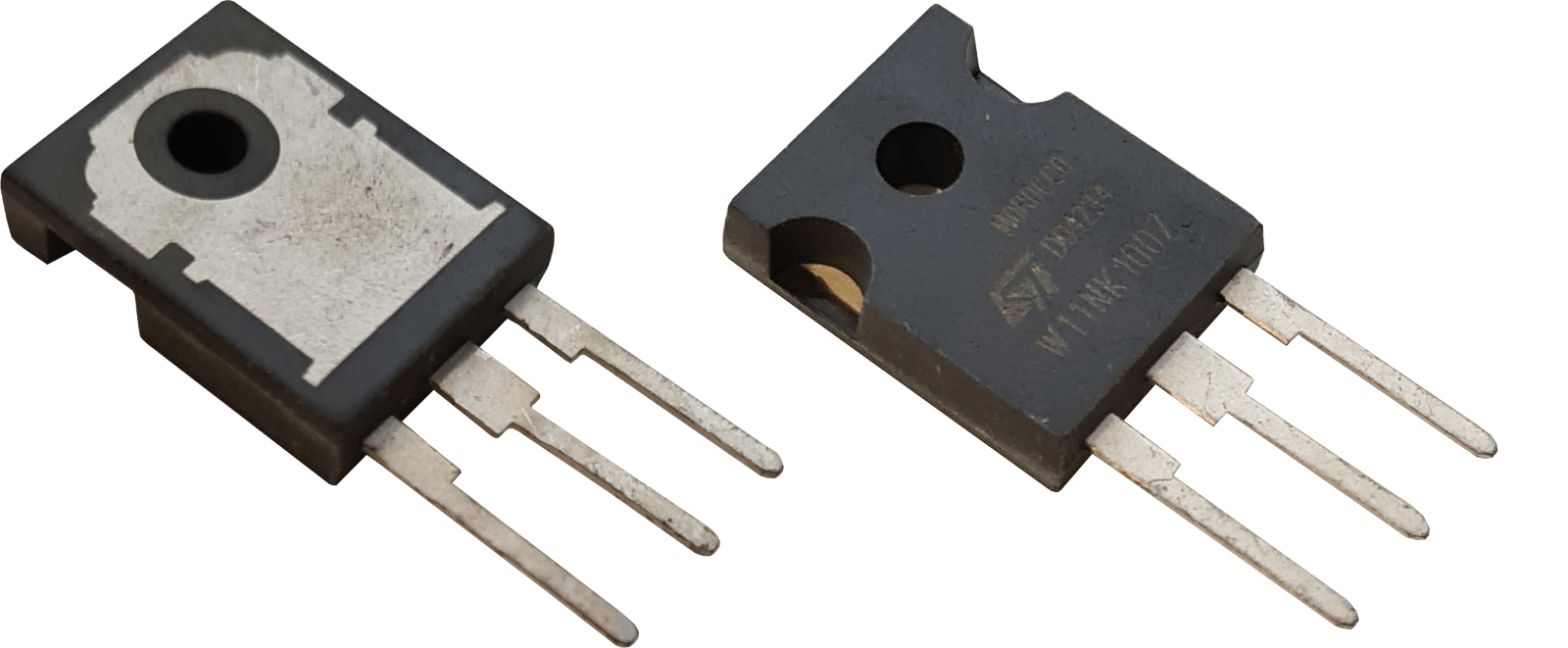
Typical N-Channel Mosfet. When using a heat sink the bare metal on the bottom of the Mosfet must be insulated using a barrier layer such as a SIL-PAD or MICA insulator
The Mosfet being used must have a voltage rating greater than your B+ voltage (preferably a lot more). The Mosfet will need to dissipate power through heat therefore a higher level of power dissipation is preferable. The current rating should more than accommodate the amp current requirements. As an example take an amp which draws 0.150A and has a B+ of 350V. The power requirement would be 0.15A x 350V = 52W. Ideally the MOSFET specification with regards to power, current and voltage should be able to accommodate the requirement multiple times over to limit any risk of overheating, shorting or failure.
MOSFETs must be handled with care during installation as they can be destroyed by static electricity. It is therefore important to handle them as little as possible and take the necessary precautions to limit any damage.
MOSFET | Details |
---|---|
2SK3675 | Vds 900V Vgs +/- 30V Id +/-7A Power 195W |
NTE2377 | Vds 900V Vgs +/- 30V Id +/-4A Power 150W |
MTP6N60E | Vds 600V Vgs +/- 20V Id +/-16.8A Power 125W |
IRF740B | Vds 400V Vgs +/- 30V Id +/-6A Power 147W |
STF9NK60ZD | Vds 600V Vgs +/- 30V Id +/-7A Power 125W |
2SK2968 | Vds 900V Vgs +/- 30V Id +/-10A Power 150W |
NTE2973 | Vds 900V Vgs +/- 30V Id +/-9A Power 275W |
IRFPG40PBF | Vds 800V Vgs +/- 20V Id +/-4.3A Power 150W Rds(on) 10V/3.5Ω |
IRFPE50 | Vds 800V Vgs +/- 30V Id +/-7.8A Power 190W |
STW11NK100Z | Vds 1000V Vgs +/- 30V Id +/-8.3A Power 230W |
STW9NK90Z | Vds 900V Vgs +/- 30V Id +/-8A Power 160W |
Vds: Maximum voltage drain to source i.e. rectified voltage.
Vgs: Maximum voltage from gate to source before risk of damage.
Id: Maximum current(A) that is supported by the MOSFET.
As at the time of writing this article the following information about vendors who provide/provided power scaling kits is available: Hall Amplification used NTE2973 for VVR, Ampmaker uses 2SK2968 for VCB, Trinity Amps use IRFPE50 for VRM.
Where to Power Scale
This is a well debated subject, subjective and down to the musicians preference. The following will cover the main areas where power scaling is applied and what impact it generally has on the behaviour and tone of the amp. When the overall power output is being reduced then other factors come into play with the overall tone and feel e.g speakers will no longer move as much air and produce their own distortions, the circuit is less likely to be starved of voltage during heavy playing and as a result reducing sag. Having an amp loud generally changes a persons mood and perception in that it makes them more positive, motivated, increases physical sensation and enhances emotions; these feelings are likely to be reduced when the amp is power scaled.
Power Section
Power section power scaling is where only the power tube voltage supply (plate and screen) is being controlled. With this type of implementation it is possible to get overdriven tones by simply reducing the voltage to the power tubes and increasing the volume to the point of overdrive. Overdrive, in this case, is generated by preamp tubes. For this type of implementation a master volume is installed (not mandatory) to control the amount of signal going into the power section and fine tune the overdrive. Master volumes can be placed either pre or post phase inverter for Push Pull or after the last preamp tube in a Single Ended amp.
Please note that there is generally only so far that the power can be reduced before the tone starts to get buzzy.
Power Section and Phase Inverter
If both power and phase inverter sections are being power scaled then installing a master volume is highly advised because overloading the PI too much will result in horrid distortion.
ExampleAn example chassis shot of cathode biased based power scaling for power and phase inverter can be seen below. Rectified B+ voltage is being fed via a yellow wire to the standby switch. It then feeds the positive connection of the reservoir capacitor (first capacitor in filter chain). Two diodes split the output; one goes to the input of the power scaling circuit and the other goes to the fourth capacitor in the filter circuit. The purpose of the diodes are to remove circuit interaction (preamp and power voltage supply circuits).
Preamp CircuitAs stated before, one of the diodes feeds the fourth capacitor in the filter circuit. The voltage in this node continues its journey to the fifth filter capacitor via a voltage dropping resistor. This final filter node feeds power to the preamp tube anodes. Because this voltage feed is not being fed through the power scaling circuit the result will be a steady voltage to the preamp tubes during power scaling (that is the intention however you may find a slight increase in voltage. This voltage increase will be inconsequential to the overall behaviour and tone of the preamp section).
Power and Phase Inverter CircuitB+ voltage exits the power scaling circuit and returns via the red wire to the turret board. There are two connections at this point 1) a red wire feeds the output transformer 2) a black wire feeds a choke and then returns to the second filter node. Two resistor from this filter node feed the screens of the EL84 power tubes. There is also a dropping resistor connecting this filter node to the next inline (3rd filter capacitor from the right). At this point a red wire connects to the phase inverter load resistors. When the voltage is reduced both phase inverter and power tubes will self bias due to them both being cathode biased. If the power tubes were fixed biased then there would be a requirement for a further mosfet and circuitry to allow bias tracking.
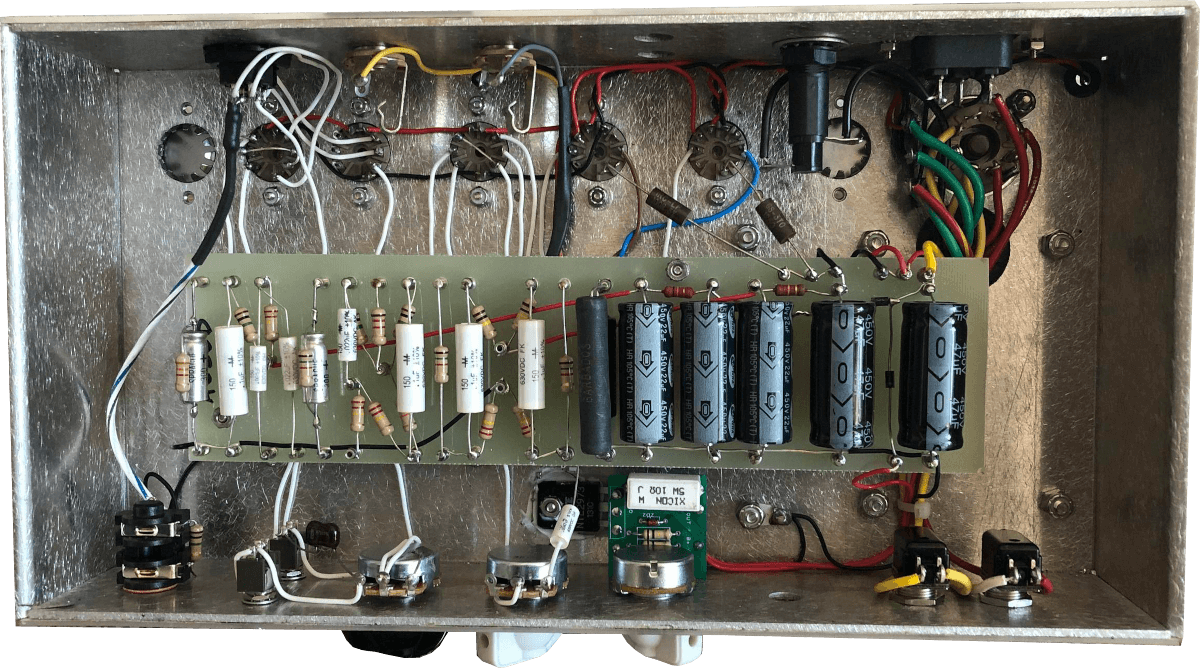
Power Tube Screen Grids
Scaling power tube screen grids only can be effective, is a simple implementation and reduces heatsink requirements for the MOSFET. Only a few mA current flow exists through screen grids therefore little heat is generated during scaling. This type of implementation is effective but can result in slightly darker tone as the screen voltages are reduced (can be counteracted by tweaking EQ). This type of power scaling can be seen in Marshall AFD100 (MOSFET STW9NK90Z) and YJM100 models, Suhr Badger. AFD100 schematic here. Suhr Badger schematic here.
Whole Amp
When the whole amp is being power scaled then there is less of a requirement for a master volume due to all gain stage voltages and output gain being reduced in sync. By including preamp tubes increase the liklehood of them producing some additional harmonic distortion. Some people have reported that including this section in power scaling has darkened the tone a little (brown sound).
One thing to note with full amp power scaling is the fact that it may cause volume pots, including guitar volume, to become scratchy. At low voltages the signal on the grids of preamp tubes leak a little DC voltage onto the guitar pot and volume control(s) when the signal hitting the grids drive them more positive than the bias voltage applied. To resolve the scratchiness a coupling capacitor can be placed before the input 1M impedance resistor or grid leak resistor following the volume of a preamp gain stage
The diagram below provides a before and after visual representation of a basic preamp layout where components are added to remove scratchiness. Components can be placed on the turret board or a tag strip for more stability. If there are any other volume pots in the preamp then there may be a requirement for further capacitors to remove scratchiness.
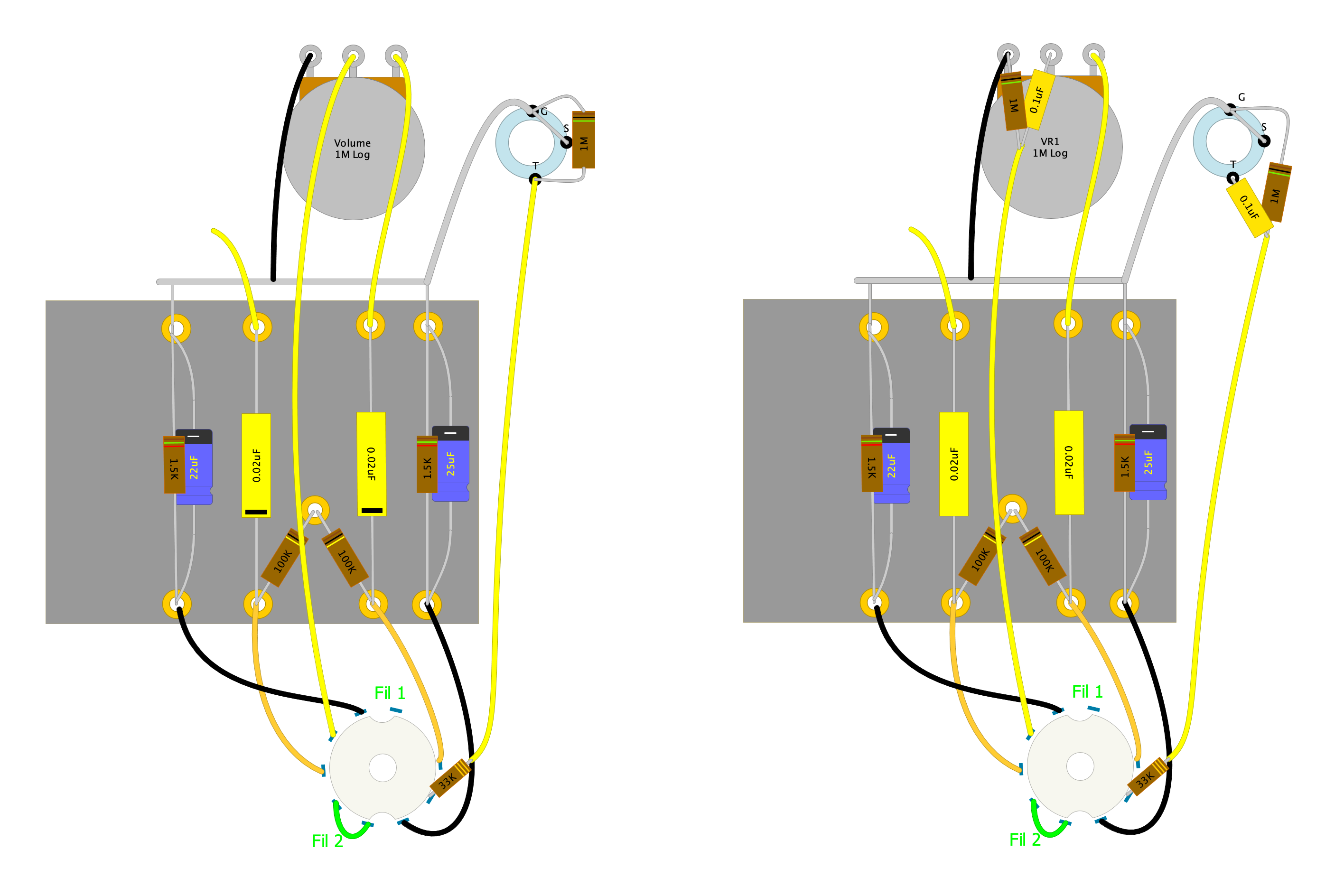
Life of Tubes
When power scaling is being used then the dissipation of tubes is reduced meaning that they are being stressed less. The result of this is the higher liklehood of the tubes lasting for longer.
Troubleshooting
Tube amplifiers contain lethal voltages even when unplugged. If you are not trained or conversant with safety measures when working with amps you should consult an amp technician.
General
Dana Hall, amongst others, have done extensive testing in power scaling circuits in many applications/amplifiers over the years. The components they provide in kit form will provide the best in performance and produce minimum issues if installed properly.
Take for example the NTE2973 MOSFET. This has been used in many commercial amplifier power scaling circuits with great success. Using a different brand or model of MOSFET may have outcomes which are not expected and/or not wanted.
Noises
A newly implemented power scaled circuit can have issues with noise. Noises can include humming, screeches and oscillation. Much like guitar amp builds things like lead dress, short runs and distance between components play an
important role in a successful implementation. Good practice for a noise free implementation is to add R-C filtering
prior to the power scaling circuit, adding a gate resistor (preferably not metal as potentially acts as an antenna) at the pin of the MOSFET, keeping wiring runs very short, keeping the circuit as far away from AC wiring as possible.
Oscillation: Oscillation can sound like a very high pitched squeal and is a result of capacitive and/or inductive coupling between inputs and outputs. This can induce a positive feedback network and as a result parasitic oscillation. MOSFETs have large transconductance (gm) and parasitic capacitances therefore
wire and any other stray conductances (between gate, source and drain circuits) can result in a positive feedback loop. When a MOSFET is in steady off or on state no oscillation occurs because its transconductance is 0 or neglible. When the MOSFET is in the linear region it is possible that
parasitic oscillations can occur. This can happen during the turning of the power scaling potentiometer or even when there are supply fluctuations causing the current protection to switch on and off (this may cause some high frequency generation which couple with other parts of the circuit to cause oscillation).
Try to identify where the oscillation is occuring by looking at the lead dress in the power scaling circuit: are lead runs long, look like spaghetti, close to AC wires? Move the power scaling
wires with a non-conductive chop stick and see if the oscillation pitch or level changes. Make sure all wires are as far away from AC wiring and are as short as possible.
Once this step has been exhausted try to make sure the 100K gate stop resistor (also part of over current circuit) is as close to the MOSFET gate as possible (preferably connected to the MOSFET pin). This will minimise stray inductances which plays a major factor in causing parasitic oscillations.
If this cannot be done then consider putting a 1K resistor (100R to 1K can be used) on the actual MOSFET gate pin and attach to the wire that returns to the 100K resistor then potentiometer mid lug. Also make sure that the zener diode is connected to the gate leg of the MOSFET or as close as possible.
Oscilliations can be induced when the drain inductance level is the same or less than the source level. It is therefore better to install a filter capacitor prior to the power scaling circuit if it is not already there. This will not only help with oscillation issues but provide first stage
filtered DC helping stabilise power scaling.
Sometimes the insulator material between the mosfet and chassis can cause stray capacitance and lead to oscillations. If silicon is installed then try a mica insulator.
Consider trying a different MOSFET. Different MOSFETs have different degrees of sensitivity within circuits. Some will be more susceptible to oscillation compared to others.
High pitched frequencies/hum: Sometimes the over current potection circuit being switched in and out causes high pitched frequencies which can interact with other parts of the circuit. The symptoms can include high pitched noises
or even hum. If the voltage drop on the sensing resistor is close to the
zener breakdown voltage then small fluctuations in current can cause this to happen. To resolve this first try replacing these components and then review the values of zener and sensing resistor and consider changing them
i.e. increase the zener voltage to something like 12V or decrease the sensing resistor value to reduce voltage drop when current flows. Do not remove the current protection circuit components as you will risk destroying the MOSFET.
Buzzing when increasing/decreasing scaling:
Make sure that there is a filter stage prior to the MOSFET so that B+ input has less fluctuation (pulsing DC) as it enters the MOSFET. Make sure there are short runs of wires between components and wires are not close to any AC wiring. Make
sure that the gate stop resistor is directly connected or as close to the gate pin of the MOSFET.
Buzzing when increasing/decreasing controls e.g. master, treble, volume:
Make sure that wires, components and ground connections in the power scaling circuit are far away from any others in the amplifier circuit. Consider moving the power scaling ground as far away from early amplifier stage ground wires and connections with the chassis.
Scratching: One thing to note with full amp power scaling is the fact that it may cause volume pots, including guitar volume, to become scratchy. At low voltages the signal on the grids of preamp tubes leak a little DC voltage onto the guitar pot and volume control(s)
when the signal hitting the grids drive them more positive than the bias voltage applied. To resolve the scratchiness a coupling capacitor can be placed before the input 1M impedance resistor or grid leak resistor following the volume of a preamp gain stage (see image below - yellow
highlights components and order).
Using a 0.1uF capcitor is less likely to impact the tone of the amp as it lets through the full frequency range of the audio signal.
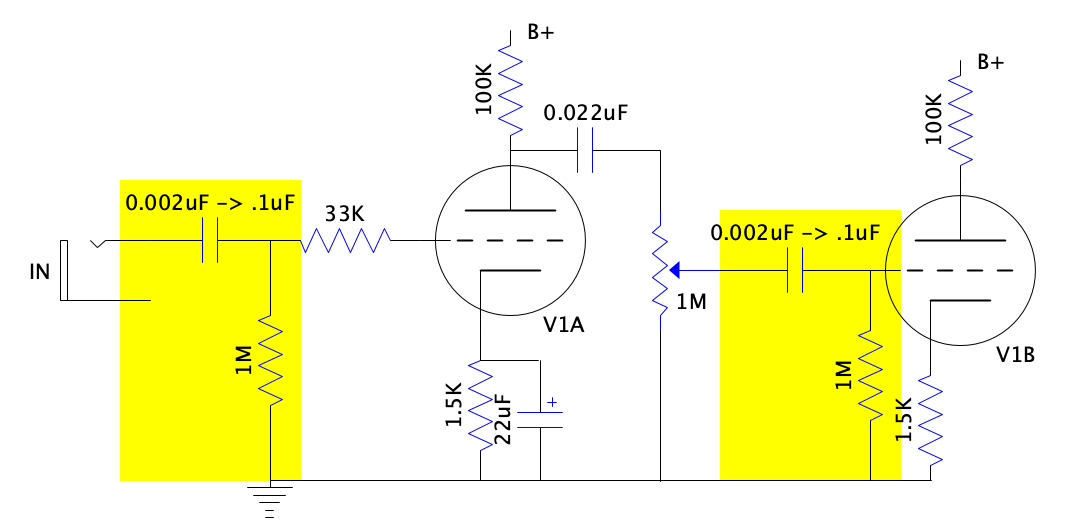
Voltage Drop
Sometimes a power scaling circuit may show a voltage drop between the drain and source of the MOSFET even when the potentiometer is set at max e.g. 340V at the drain pin and 310V at the source pin. This can happen when the current limiting circuit starts to kick in. Triggers of current limiting include an inrush of current (capacitors charging on startup, tubes and/or components drawing large amount of current), too small a breakdown voltage for the zener diode in the context of the circuit or a faulty zener diode. If there is a 6V zener installed in the circuit then consider changing this to a higher value such as 12V; alternatively if you have a 10R current sense resistor in place then consider changing to 5R. Do not raise the zener value much higher as you need to make sure you do not reach the limits of the MOSFET (Vgs) and risk damaging it. Also consider rolling power tubes to see if they reduce the circuit current requirements and as a result stop the current limiting from kicking in.
Double check that the circuit does not have a B+ limiting resistor (to set B+ max voltage) in the circuit. In the diagram below the 330K resistor sets the maximum B+ voltage and the 47K resistor sets the minimum.
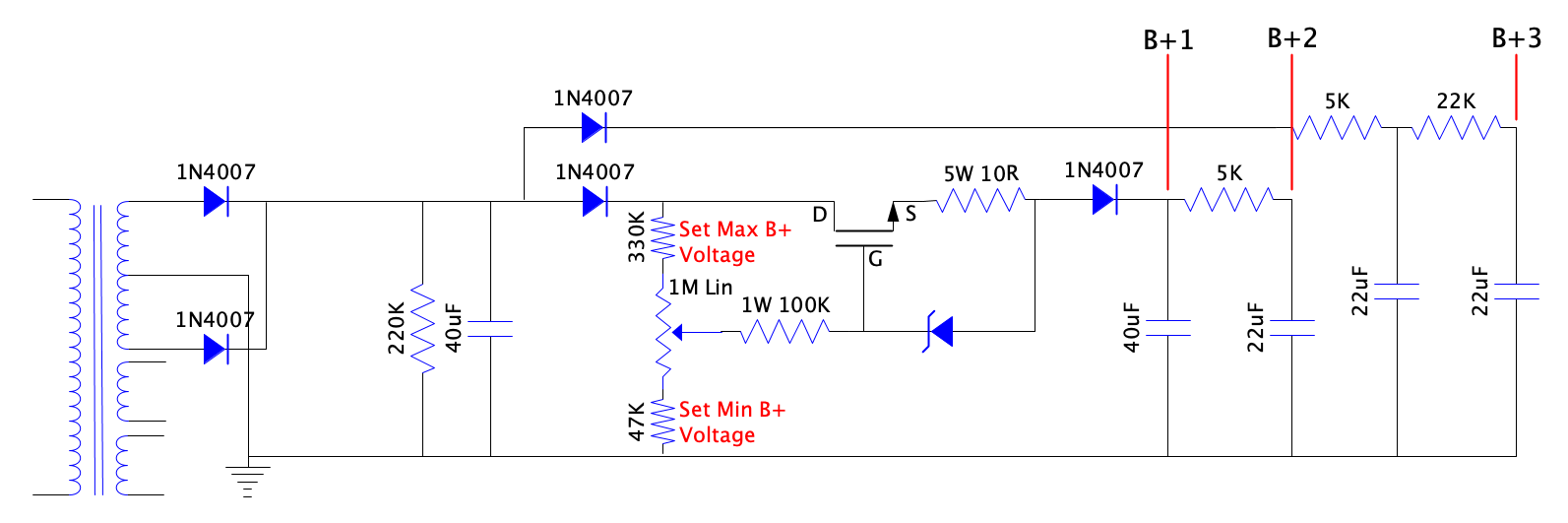
Blowing MOSFET
Make sure that there is a filter stage prior to the MOSFET so that B+ input has less fluctuation (pulsing DC) as it enters the MOSFET. Make sure that the current limiting circuitry is connected properly and of correct value i.e. zener in correct orientation, sensing resistor has the correct resistance. Check that the MOSFET power handling, maximum voltage / current / gate-source voltages can accommodate the amplifier having the installation. Check for shorts to chassis in the wiring. Make sure that the MOSFET is not shorting to the chassis (if attached to the chassis) - insulated via a silipad or mica; nylon bolt where applicable.
Reverb and/or Tremolo intensity decreasing
If you have implemented whole amp power scaling or power amp where one of the nodes being scaled feeds reverb and/or tremolo then it is likely that the effect will decrease or even stop when reducing the voltage. To not effect reverb or tremolo there will be a requirement to not scale the voltage for the feed to these parts of the circuit. This may require that you take a voltage feed at the rectifier, filter and reduce the voltage to the expected value before directing to the reverb or tremolo circuitry. The alternative is to limit the power scaling to only the plate and screens of the power tubes.
Reduced Bass and Treble
Human hearing reacts differently to low versus high listening volumes. At low listening volumes mid range is more prominent while low and high frequency ranges go into the background. At higher listening volumes the lows and highs sound more prominent whereas the mid range is softer. Loss of lows and highs can be compensated by turning up the bass and treble controls or implementing a boost of bass and treble either via a switch or as part of the power scaling circuit.